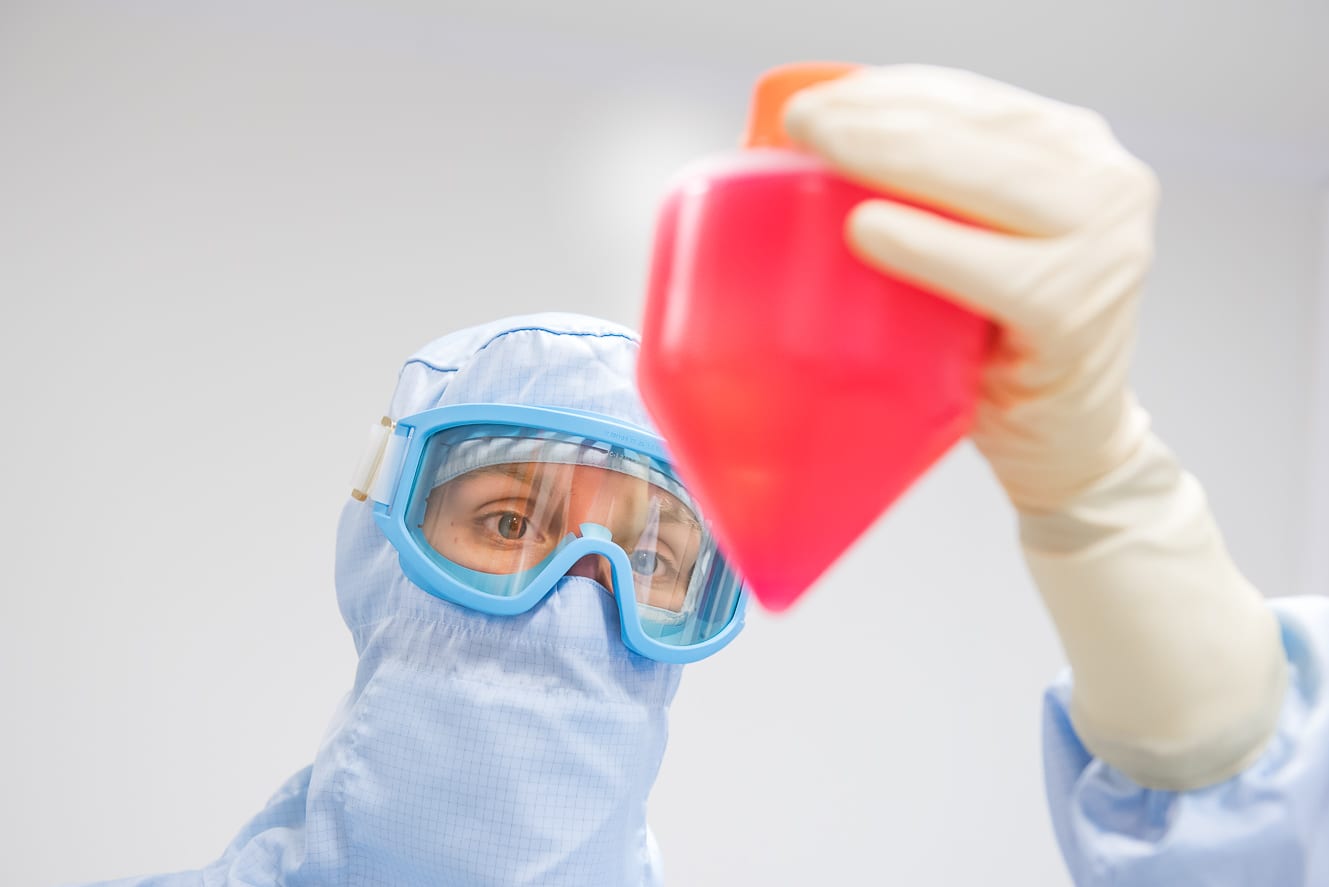
Development of the Next Generation NKG2D CAR T-cell Manufacturing Process
Celyad’s view on developing and delivering a CAR T-cell therapy with multi-tumor specificity combined with cell manufacturing success
Overview
Transitioning potential therapeutic assets from academia into the commercial environment is an exercise that is largely underappreciated by stakeholders, except for drug developers themselves. The promise of preclinical or early clinical results drives enthusiasm, but the pragmatic delivery of a therapy outside of small, local testing is most often a major challenge for drug developers especially, including among other things, the manufacturing challenges that surround the production of just-in-time and personalized autologous cell therapy products.
While developing a series of NKG2D chimeric antigen receptors (CARs), which Celyad believes carry enormous potential through their breadth of potential tumor targeting, creating solutions to ensure the manufacturability and stability has required further examination of the basic biology around the CAR and its targets. This detailed knowledge has given rise sequentially to several potential manufacturing solutions within a few months. The hurdles of low yield and lack of product stability following cryopreservation were major challenges that Celyad has overcome to generate what it believes is a robust manufacturing process for its series of NKG2D-based CAR product candidates. Continuing in-house research has sought further improvements to the process, especially focusing on generating less differentiated T-cells which are currently considered to be optimal for generating an adoptive CAR T-cell therapy with potential long-lasting impact. This advancement led rapidly to our current proprietary manufacturing process called ‘OptimAb’, which is now implemented in the company’s two different clinical autologous NKG2D-based CAR product candidates, CYAD 01 and CYAD-02.
CAR T-cell Therapy
Early beginnings and limitations of the single targeting classical construct
The concept of the chimeric antigen receptor (CAR) was developed by Zelig Eshhar at the Weizmann Institute, Israel during the late 1980s and early 1990s. The CAR approach was developed specifically in response to observations that tumor cells actively avoid immune-mediated elimination by the specific downregulation of crucial molecules that mediate immune recognition. T-cells are key effectors of the immune system that use a ‘T-cell receptor’ to bind to the major histocompatibility complex (MHC) present on every nucleated cell. Tumor cells actively interfere with MHC which, in effect, results in the tumors becoming invisible to T-cell surveillance. This is a major reason why tumors can grow despite the presence of T-cells that could readily eliminate the tumor.
The CAR approach involves engrafting T-cells with a novel cell surface receptor that provides a tumor specificity and is independent of the usual T-cell recognition system. In the most common iteration, this receptor is based on an antibody which can directly recognize target proteins on the cell surface in the absence of MHC, and thus remove the cloak of invisibility from the tumor and expose them to T-cell mediated killing.
CAR T-cell therapy is now a clinical reality having moved from early concept to clinical proof of principle and, in 2017, to the U.S. licensing of two CAR T-cell products for the treatment of B-cell malignancies. The rapid approval of the two products Yescarta™ and Kymriah™ were based on clinical responses in patients for whom there were little, if any, therapeutic option that could drive long-term benefit. These approvals were groundbreaking for the industry and for the treatment of cancer. However, the expectation of a flood of CAR T-cell therapies beyond B-cell malignancies delivering similar levels of clinical efficacy has not yet been fulfilled. We believe this is largely due to several issues, which include suitability of targets with which to aim the CAR T cell to tumors and the challenge of manufacturing CAR T-cells in a commercially viable manner that is able to supply the need of the worldwide addressable patient population.
The potential advantage of using antibodies for CAR T-cell therapy is their exquisite specificity for a single target. The target typically determines the indication for which the CAR T-cell therapy can be used. Yescarta™ and Kymriah™ both use an antibody specific for the CD19 protein present on B-cell leukemias and lymphomas. Since CD19 is absent on non-B-cells, these two products cannot be effectively used beyond treating B-cell malignancies. Outside of B-cell tumors, there are unfortunately very few ideal targets similar in profile to CD19. The advantage of mono-target specificity of antibodies is also potentially a disadvantage since the tumor may lose the specific target (a process called ‘antigen loss’) and hence become effectively invisible to the CAR T-cell therapy. This is indeed a phenomenon appearing within CD19 CAR T-cell product candidates, where patients are succumbing to B-cell leukemias and lymphomas despite the presence of CD19 CAR T-cells in their blood, as the tumor blasts have lost the CD19 protein.
Finding a system that provides tumor specificity and overcomes tumor antigen escape by targeting multiple tumor specific epitopes may be a successful combination to deliver a broad CAR T-cell therapy approach.
NKG2D
A possible answer to the broad CAR T-cell therapy conundrum
An obvious answer to multiple tumor targeting for CAR T-cells is to use multiple antibodies and receptors (Springuel et al. 2019)¹. This somewhat manual approach is indeed being explored extensively in the CD19 CAR T-cell space in response to antigen-loss variants seen after CAR T-cell therapy. However, a potentially more exquisite approach would be to use multi-target specificity designed by nature itself. Natural killer (NK) cells are a part of the innate immune system that has evolved to provide an immediate response to infection by pathogens such as viruses. One specific mechanism employed by NK cells is through the Natural Killer Group 2D (NKG2D) receptor which binds to eight known targets in humans. These targets are ‘stress ligands’ that are displayed by cells rapidly after microbial infection, among others. Thus, this single NKG2D receptor enables NK cells to recognize any of the eight targets displayed by infected cells and initiate a killing response. In this manner, escape of NKG2D-mediated surveillance would likely require loss of all eight targets (Figure 1).
Importantly, many tumors (both hematological and solid) display these NKG2D targets mainly as a result of genome instability. However, tumors avoid NK cell mediated killing by many factors including the fact that NK cells are only rarely found within the tumor environment. Professor Charles Sentman² conceived the idea of using the NKG2D receptor fused to a T-cell activation domain to form a CAR. When expressed in a T-cell, this receptor could potentially provide an approach that is truly broad (in targeting a wide array of tumors) and aimed at multiple targets (thereby potentially reducing antigen-escape variants to develop).
Figure 1: Comparison of single-target and multi-target CAR T approaches
Click on the image to see the full-sized version
Extensive preclinical studies observed the potential of a NKG2D-based CAR T-cell investigational therapy, which progressed into initial Phase 1 clinical testing at the Dana Farber Cancer Institute in 2015. The academic mono-centric CM-CS1 trial used low doses of fresh NKG2D CAR T-cells produced at an on-site cell production facility. Twelve patients with advanced multiple myeloma or acute myeloid leukemia received NKG2D CAR T-cells in this dose-escalation safety trial. Given the breadth of target binding, the key readout from this trial was that the CAR T-cell investigational therapy was well tolerated with no evidence of on-target, off-tissue toxicity (Baumeister et al. 2019)³.
Armed with initial clinical safety data and the therapeutic potential of the NKG2D-based CAR T-cell investigational therapy, Celyad decided to actively pursue the approach into larger scale, industry-sponsored clinical trials to investigate the safety profile of the investigational cell therapy and to assess the clinical activity of the NKG2D-based approach.
Transitioning from Academic to Larger Cell Therapy Trials
Logistics and manufacturing challenges
One important challenge facing Celyad was related to moving into multicenter, multinational clinical trials for the NKG2D CAR T-cell investigational therapy that employed multiple doses of autologous patient’s T-cells produced from a single apheresis. This required the cell product to be frozen at the end of the manufacturing process, for shipment of the several doses to the treating centers all around the world. The cells are then stored frozen and thawed just before the patient is treated (Figure 2). The company strongly benefited from its history in cell therapy manufacturing and expertise gained from its own bespoke cell manufacturing facility. Moreover, the company also possessed in-house experience in producing cell therapy product candidates for large multicenter clinical trials, meaning that many of the logistical hurdles that hinder cell therapy, including international (and indeed, intercontinental) cold-chain delivery, had already been addressed by the company.
Figure 2: Schematic representation of the manufacturing process of a fresh or frozen CAR T-cell product.
Click on the image to see the full-sized version
However, while the fundamentals of delivering an investigational cell therapy to clinical sites was largely already established in-house, it was clear at a very early stage that the academic manufacturing process employed for the CM CS1 clinical trial to deliver a fresh dose of NKG2D CAR T-cells should be adapted for the production of multiple doses of frozen CAR T-cells. Firstly, the number of cells that could be produced was limited and, secondly, the freezing of cells (cryopreservation) resulted in a significant loss of living NKG2D-based CAR T-cells. Both are major restrictions to delivering a robust just-in-time manufacturing solution for patients with advanced cancer.
Figure 3: Fratricide effect after thawing of NKG2D CAR T cells
Click on the image to see the full-sized version
The reasons for low cell number and poor viability after cryopreservation appeared linked. Our research showed that the targets for the NKG2D CAR are transiently expressed on the activated T-cells meaning that that T-cells armed with the NKG2D CAR could potentially kill other T cells, and possibly themselves, during the culture period (termed fratricide). More concerning, these NKG2D targets are increased during cellular stress and the freeze-thaw process induces stress that results in the major upregulation of NKG2D targets and thus explains the rapid loss of viable T-cells after thawing through fratricide (Figure 3). Dealing with these two issues has been a challenge for the company to overcome over the past few years. During that time our manufacturing has been through different iterations resulting in a process that is able to supply all current clinical activity with high manufacturing success rates in a commercially relevant context.
Process Development
An essential, continuing improvement exercise
Another often overlooked concern is the fact that some assets brought into the company come with fundamental issues around manufacture. However, rather than shy away from the challenge, the research teams at Celyad focused upon exploring pragmatic answers to the issues. The first solution focused upon cryopreservation and the serendipitous observation that certain kinase inhibitors could enhance the recovery of NKG2D CAR T-cells after thawing. The inclusion of a phosphatidylinositol 3-kinase (PI3K) inhibitor (LY294002, referred to as LY) into the manufacturing process significantly enhanced cell yield (Figure 4). The first clinical trial initiated by Celyad (THINK trial, NCT03018405), aimed to evaluate the frozen NKG2D CAR product candidate (CYAD-01) in a multiple infusion schedule with no prior preconditioning (three to six doses of cells with up to 3 billion cells per infusion). All doses for a specific patient were produced from a single apheresis and were then frozen and delivered to the clinical site for infusion over a one-month period. The inclusion of LY proved to be a relatively minor addition to the academic manufacturing process but one that enabled the initiation of the THINK clinical trial in clinical centers in the EU and U.S. in early 2016.
Figure 4: Reduction of the fratricide effect through implementation of the LY and mAb processes
Click on the image to see the full-sized version
As the trial progressed, the issue of cell yield became more pressing. Producing enough cells from patients with advanced hematological malignancies was problematic. We believe the reasons for this poor yield were again likely due to the fratricide issue. Our continued research identified that the inclusion of a NKG2D-blocking monoclonal antibody (anti-CD314) to block the fratricide effect resulted in high cell yields and much improved consistency of production. The inclusion of the monoclonal antibody into the manufacturing process (commonly referred to as the mAb process, Figure 4) again was a fairly minimal amendment but resulted in a highly reproducible and robust process that, since introduction in January 2018, has resulted in a greater than 94% manufacturing success rate for the just-in-time autologous approach.
OptimAb Process
Moving process development beyond increasing the yield
The generation of a robust manufacturing process that overcomes the challenges of fratricide is designed to enable the production of sufficient numbers of cells for all of Celyad’s NKG2D CAR T-cell trials. However, research continues and, recently, rather than dealing with overcoming pragmatic issues, has focused on efforts to generate cells that may have increased anti-tumor activity in the patient. This is of importance to Celyad given the company’s current focus on the highly challenging clinical indications of advanced acute myeloid leukemia and metastatic colorectal cancer.
There is an emerging view in the cell therapy field that T-cells that display features associated with reduced differentiation may be more active in the therapeutic setting. Mature T-cells are derived from progenitor cells that are produced in the bone marrow and mature through a process of ‘education’ in the thymus. The T-cell that emerges from the thymus is known as a naïve T-cell and circulates the body looking for action. This action takes place in secondary lymphoid structures such as lymph nodes and it is here where the naïve T-cell meets its target and becomes licensed to go out and kill. Eventually, the T-cell leaves the lymph node to do its business of chasing infected and cancer cells around the body. As it does this, the T-cell becomes more differentiated – in short, the T-cell when first made is naïve or de-differentiated and designed to move around the body in order to go to the correct structures to become educated. As the T-cell emerges to deal with challenges around the body, it becomes more mature and set in its ways – this is differentiation. As T-cells become more differentiated, they are better able to kill target cells, but this is also associated with a shortened life span – they tend to die during the process of killing. This is a natural mechanism used to ensure that highly powerful and destructive T-cells do not persist for excessively long periods of time which could result in toxicity.
With respect to CAR T-cell investigational therapy, the manufacturing processes used to date tend to produce more differentiated T-cells that are highly active in killing but less able to persist. Studies in mouse models suggest that generating less differentiated T-cells may deliver a stronger anti-tumor effect. This is a little counter-intuitive since less differentiated T-cells possess less direct cancer cell killing activity, but this can be counterbalanced by the fact that this less active killing persists for long periods.
Translating the observations made in mice to human clinical studies is not straightforward. However, our in-house research suggested that swapping the inhibitor used in our manufacturing process for a different, more specific inhibitor and reducing the length of culture from ten to eight days produced cells that had a higher frequency of a protein on their cell surface that indicated the cells were less differentiated. In more detail, this altered process (now called OptimAb) generated a NKG2D CAR T-cell population with a higher frequency of CD62L (or L-selectin) on the cell surface as compared to those generated by the mAb process (Figure 5). CD62L is a protein used by T-cells to traffic to lymph nodes; thus, this protein is present on cells that are less differentiated and tracking to lymph nodes. Consequently, T-cells that express CD62L are likely to be less differentiated. However, we have also observed in mouse models that OptimAb T-cells also produce higher level of interferon gamma upon challenge with tumor cells, a cytokine which plays an important role in driving anti-tumor effect.
Figure 5: Comparison of the mAb and OptimAb manufacturing processes
Click on the image to see the full-sized version
In a stress-test mouse model, when comparing mAb-produced NKG2D CAR T-cells (at a reduced dose to achieve a minimal level of anti-tumor activity), the OptimAb NKG2D CAR T-cells showed much improved anti-tumor activity at the same dose. Taken together with other internal data, these observations suggested that through another relatively small change of the manufacturing process, NKG2D CAR T-cells with potentially increased potency could be produced. This OptimAb manufacturing approach is supported by the clinical tolerability of NKG2D CAR T-cells observed in several Phase I clinical trials. Therefore, the decision to produce T cells with the same specificity profile but likely improved function in line with the current literature was strongly warranted.
Our proprietary OptimAb process was accepted by both the U.S. Food and Drug Administration (FDA) and the Federal Agency for Medicines and Health Products (FAMHP) of Belgium as an amendment to the manufacturing process for current clinical trials of investigational NKG2D CAR T cells.
“We eagerly await the translational outcomes of our efforts to control and now improve the manufacturing of investigational NKG2D CAR T-cells.”
David Gilham, VP Research & Development, Celyad
¹ Springuel et al. 2019. “Chimeric Antigen Receptor-T-cells for Targeting Solid Tumors: Current Challenges and Existing Strategies.” BioDrugs. https://doi.org/10.1007/s40259-019-00368-z.
² Sentman and Meehan. 2014. Cancer J. 2014 Mar-Apr;20(2):156-9.
³ Baumeister et al. 2019. “Phase I Trial of Autologous CAR T-cells Targeting NKG2D Ligands in Patients with AML/MDS and Multiple Myeloma.” Cancer Immunology Research 7 (1): 100–112.